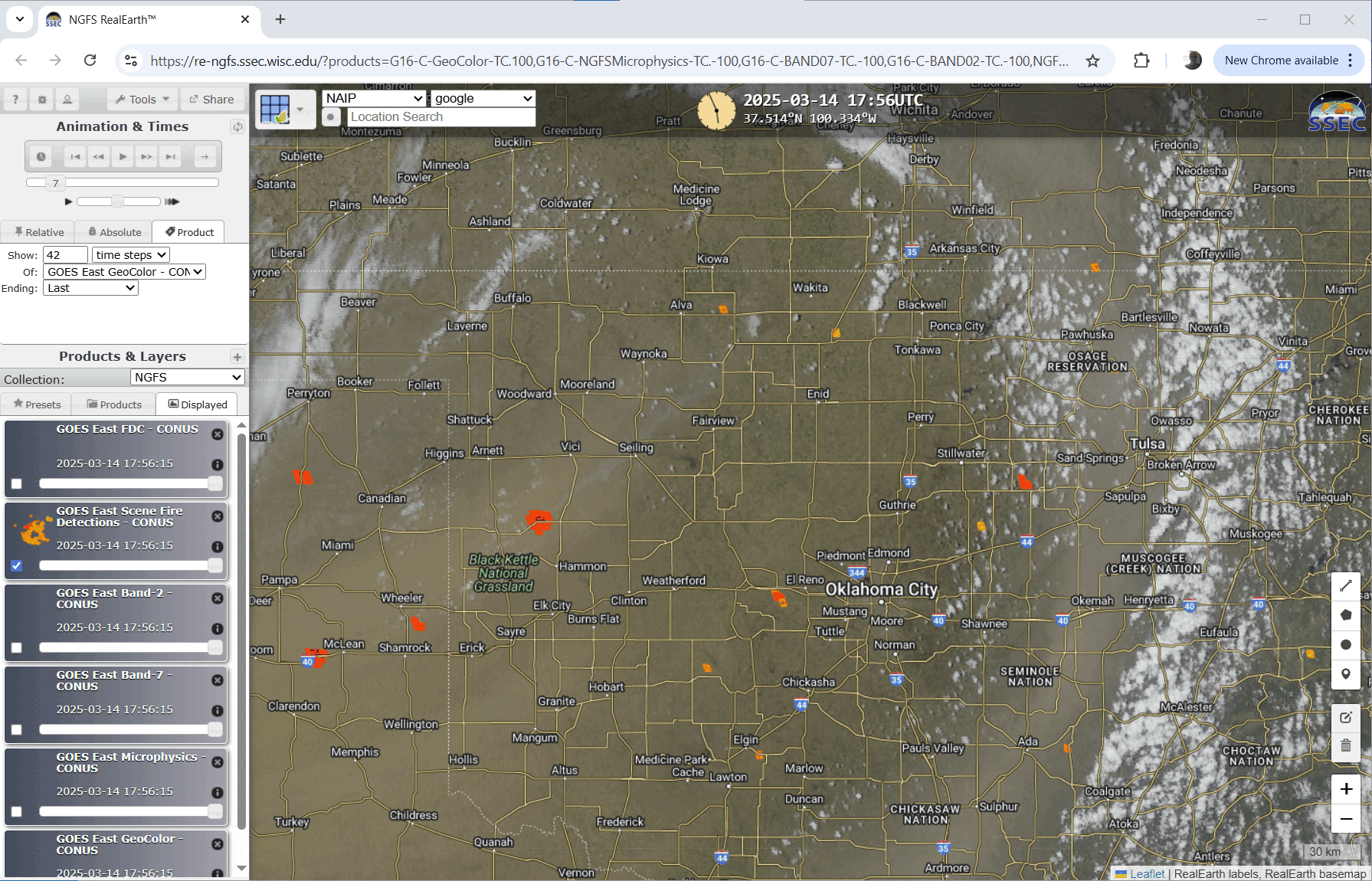
Next-Generation Fire System (NGFS) data within a RealEarth instance (direct link), above, shows the rapid development and growth of multiple fires during the day on 14 March 2025. The RealEarth instance might be an easier way to track fires on an Extreme Fire Weather day like 14 March; the NGFS Alerts Dashboard (https://cimss.ssec.wisc.edu/ngfs) below (showing only detections in Texas and Oklahoma), in a screenshot from just after 0000 UTC on 15 March), shows many many detections and unless you are keenly aware of Oklahoma geography and county names, it’s hard to know at a glance which fires are which. When plotted on a map as shown above, they are easier to track.
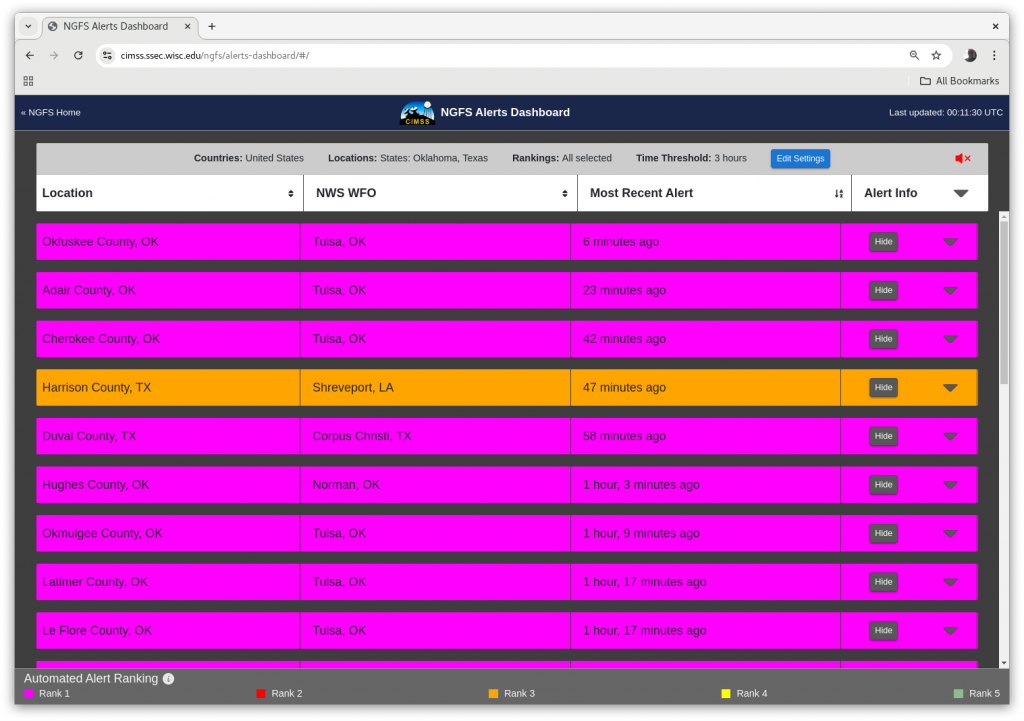
The RealEarth instance has imagery that is probe-able. The probe shown below of the Hickory Hill Road fire lists out different satellite-derived properties at the point probed.
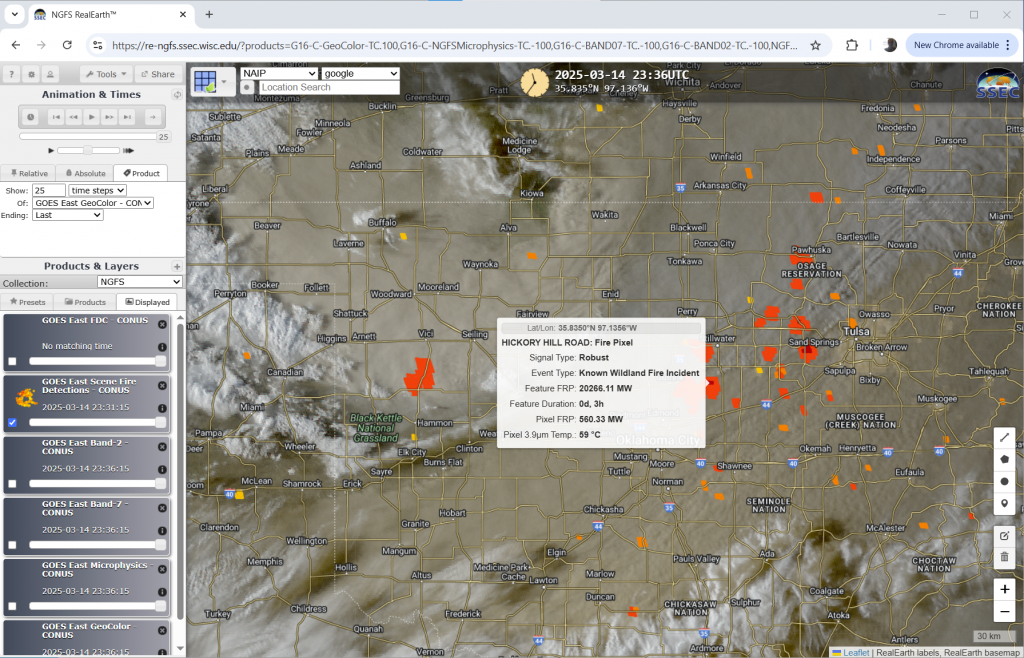
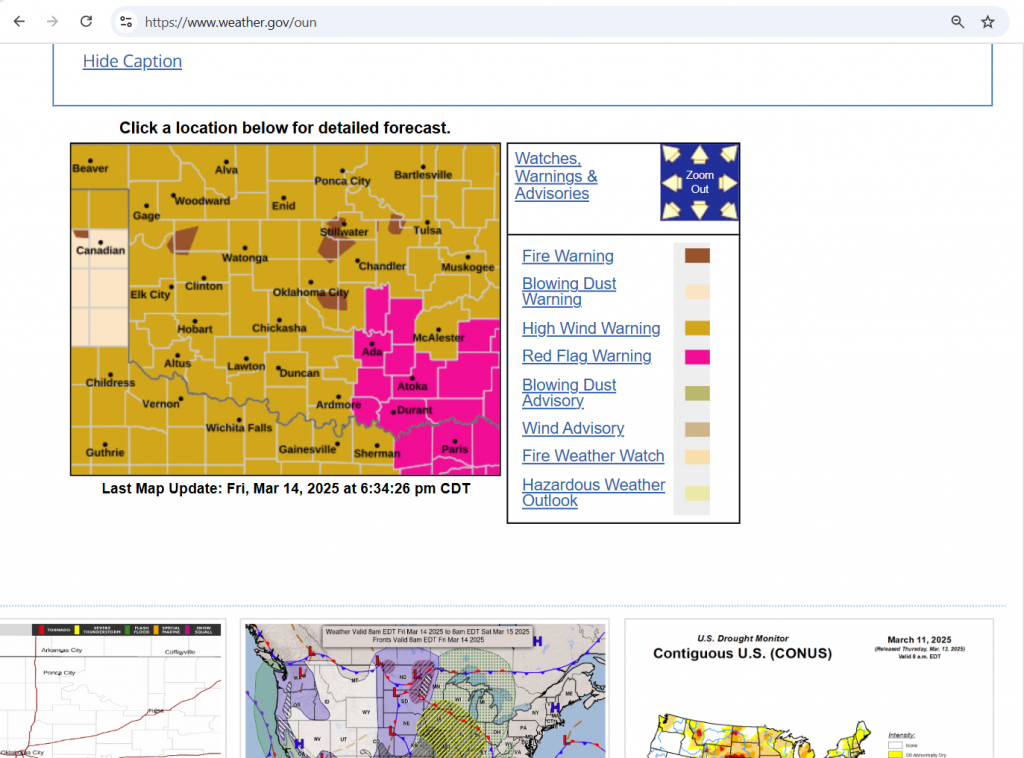
Multiple Fire Warnings have been issued over Oklahoma. At 2336 UTC, the Norman OK WFO website (above) showed the location of active warnings. The NGFS RealEarth display for that time is shown below. Not all of the fires indicated at that time below have Fire Warning associated with them. A Fire Warning occurs as a request to the NWS Forecast Office by their partners. For example, if you were to click on the map above within the Fire Warning polygon southwest of Stillwater, you would have access to the Fire Warning text shown at bottom, issued at the request of the Oklahoma Forestry Service.
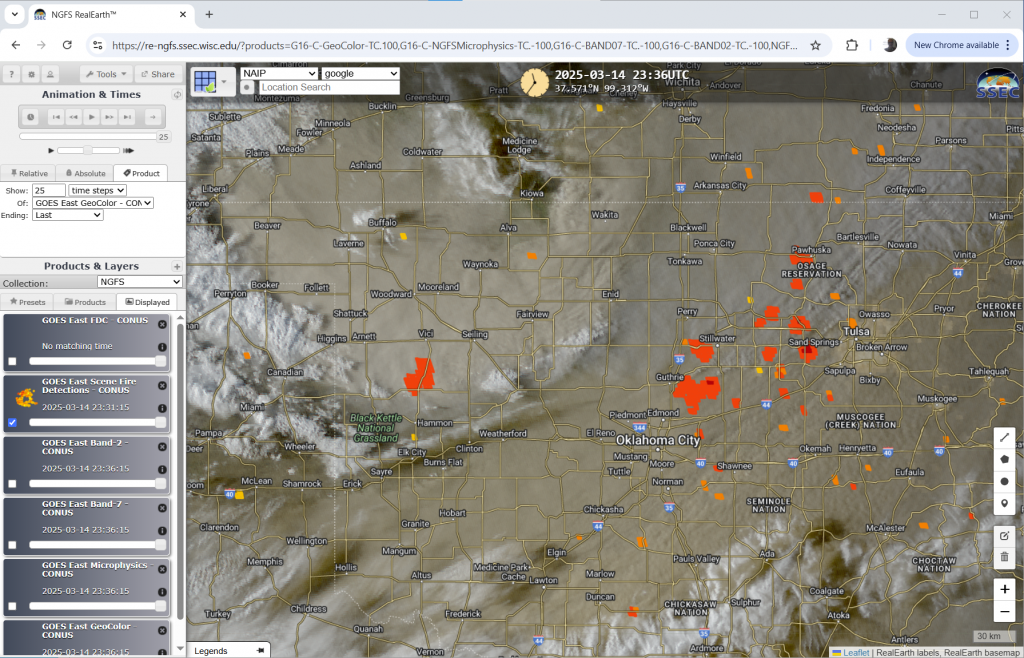
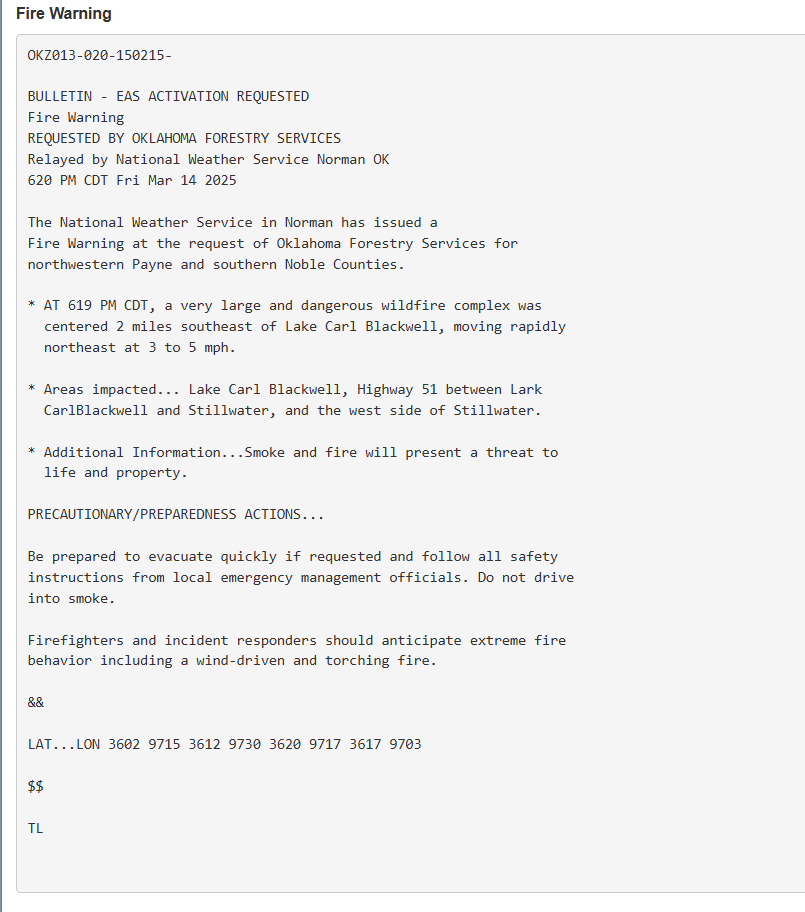
The graphic below, from WFO OUN, testifies to the historic nature of this wildfire outbreak. The extraordinary winds — Stillwater OK airport saw gusts exceeding 70 knots — are helping to drive the fires. The widespread dust is apparent in the RealEarth imagery above
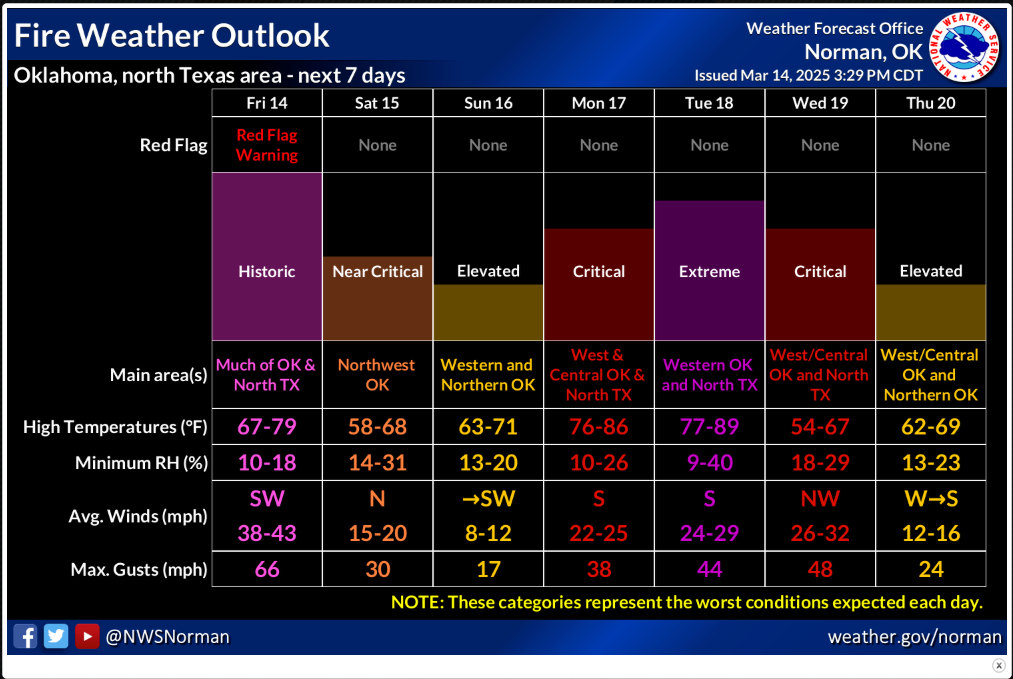
There was a very timely and informative Satellite Book Club presentation on Wildfire operations on 13 March 2025. Click here to listen. A separate CIMSS Blog Post (here) discusses the widespread blowing dust event that occurred as the fires burned.
View only this post Read Less