10-minute Full Disk sector GOES-18 (GOES-West) Day Land Cloud Fire RGB, Shortwave Infrared (3.9 µm), “Clean” Infrared Window (10.3 µm) and “Red” Visible (0.64 µm) + Fire Power derived product (a component of the GOES Fire Detection and Characterization Algorithm FDCA) images (above) showed signatures of multiple wildfires across northeastern British Columbia, two of which produced pyrocumulonimbus (pyroCb) clouds — having cloud-top... Read More
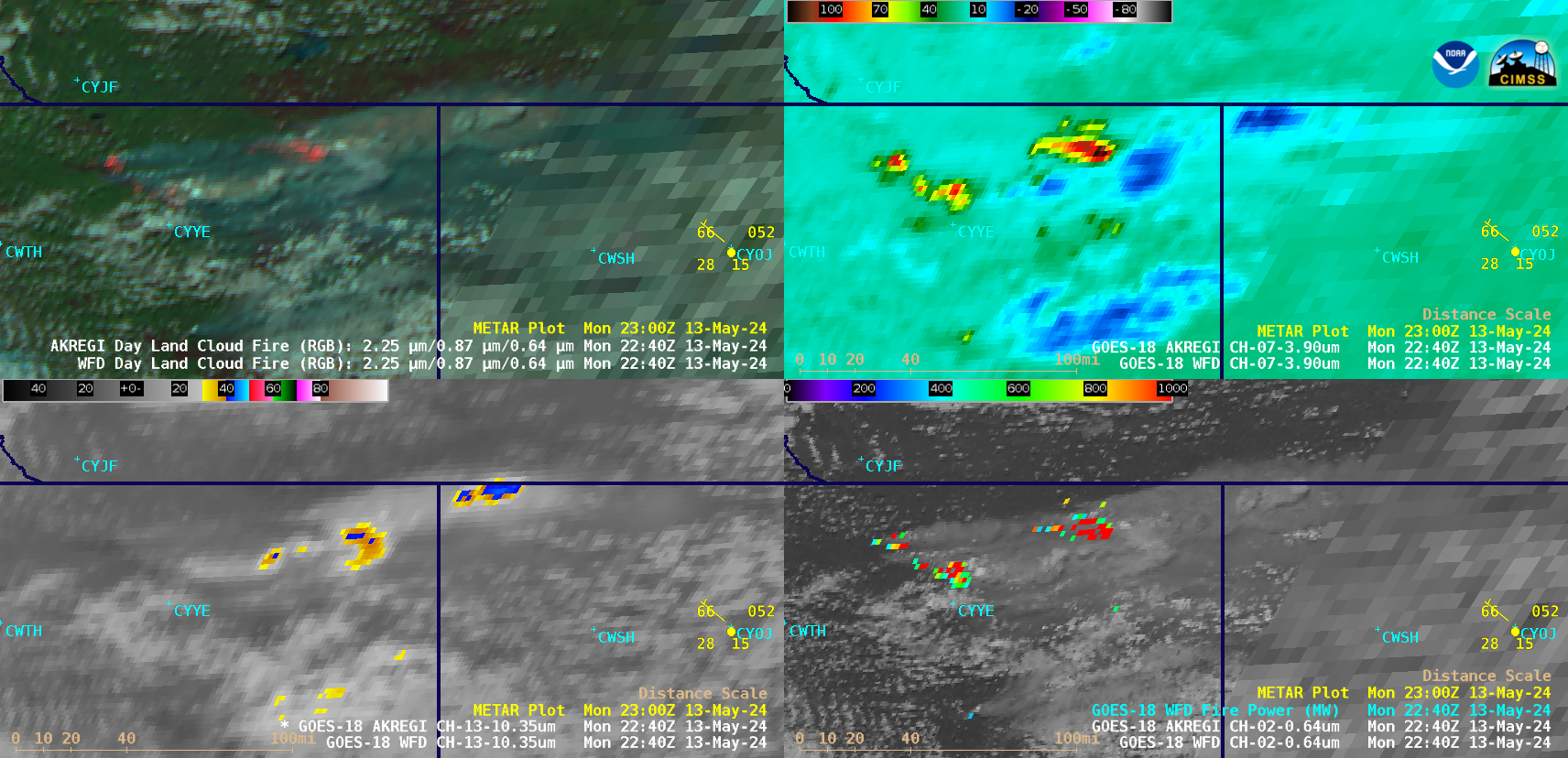
GOES-18 Day Land Cloud Fire RGB (top left), Shortwave Infrared (3.9 µm, top right), “Clean” Infrared Window (10.3 µm, bottom left) and “Red” Visible (0.64 µm) + Fire Power derived product (bottom right), from 1900 UTC on 13 May to 0040 UTC on 14 May [click to play animated GIF | MP4]
10-minute Full Disk sector GOES-18
(GOES-West) Day Land Cloud Fire RGB, Shortwave Infrared (
3.9 µm), “Clean” Infrared Window (
10.3 µm) and “Red” Visible (
0.64 µm) + Fire Power derived product (a component of the GOES Fire Detection and Characterization Algorithm
FDCA) images
(above) showed signatures of multiple wildfires across northeastern British Columbia, two of which produced
pyrocumulonimbus (pyroCb) clouds — having cloud-top infrared brightness temperatures of -40ºC and colder, denoted by shades of blue in the 10.3 µm images — late in the day on 13 May 2024 (these were Canada’s first pyroCb clouds of their 2024 wildfire season, which has gotten off to an unusually early start). Wildfire smoke drifting eastward was intermittently reducing the surface visibility at High Level, Alberta (CYOJ).
The largest of these fires burned very hot, exhibiting 3.9 µm shortwave infrared brightness temperatures of 137.88ºC (the saturation temperature of GOES-18 ABI Band 7 detectors) — with Fire Power values intermittently exceeding 6200 MW (below).
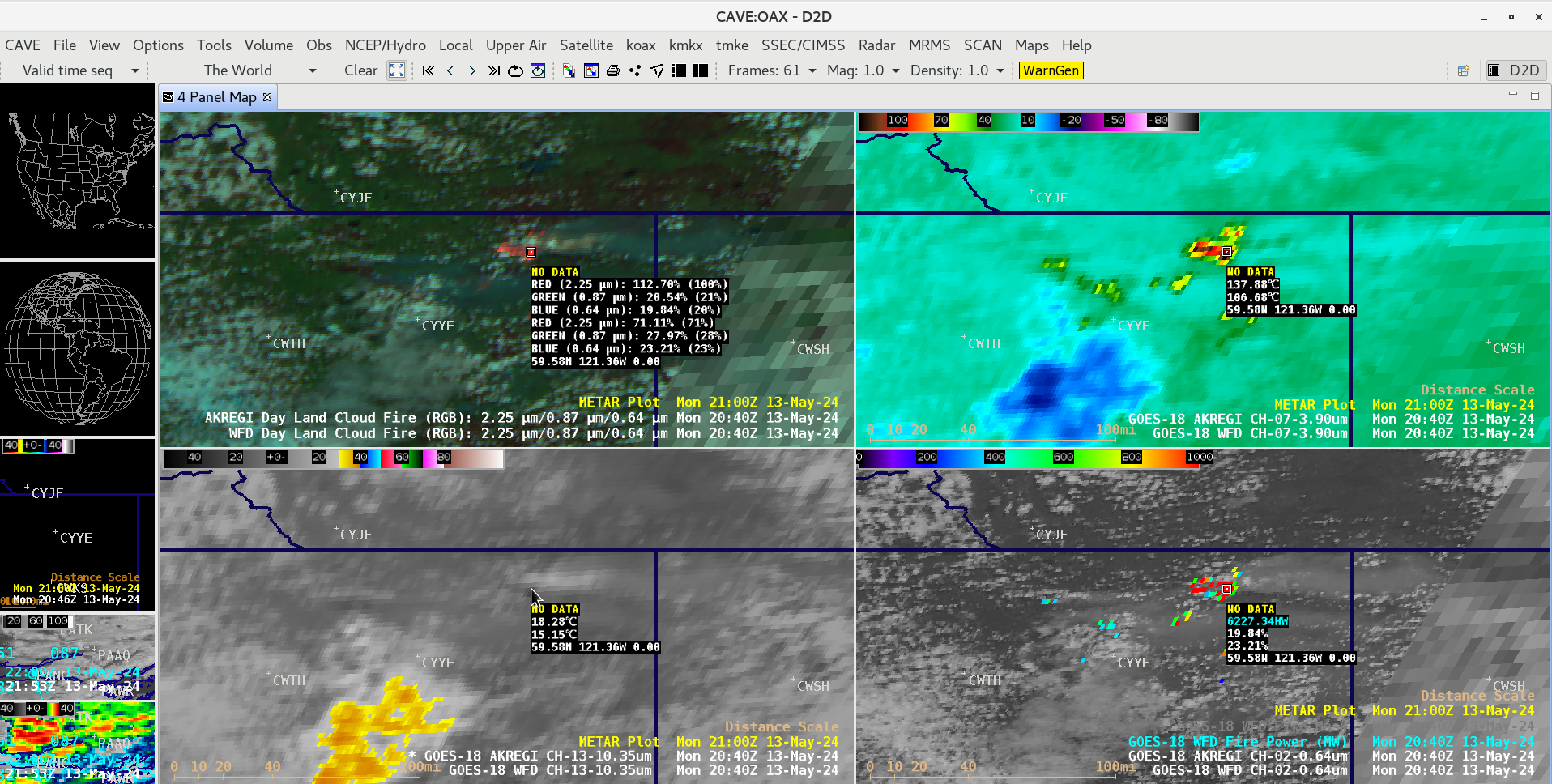
Cursor sample of GOES-18 Day Land Cloud Fire RGB (top left), Shortwave Infrared (3.9 µm, top right), “Clean” Infrared Window (10.3 µm, bottom left) and “Red” Visible (0.64 µm) + Fire Power derived product (bottom right) at 2040 UTC on 13 May [click to enlarge]
GOES-18 True Color RGB images from the
CSPP GeoSphere site
(below) displayed the dense plumes of wildfire smoke, with pyroCb clouds produced by the larger, more intense wildfires rising above the tops of the smoke (casting shadows onto the smoke layer below).
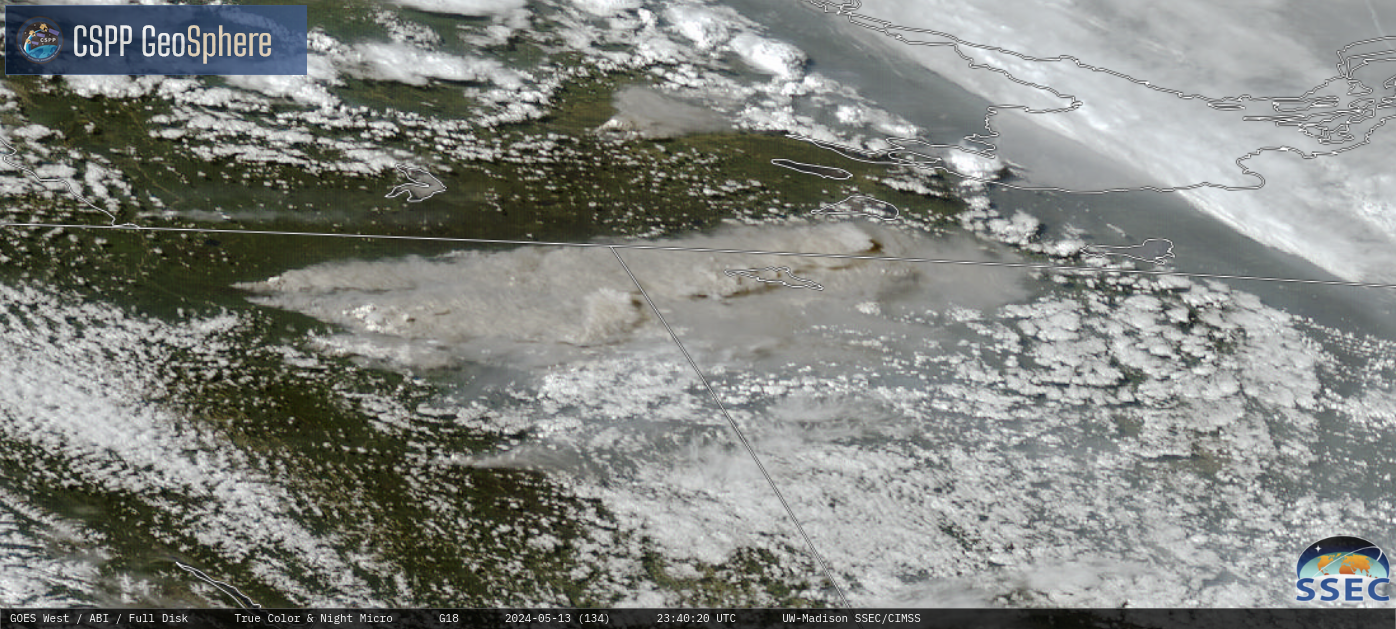
GOES-18 True Color RGB images, from 1600 UTC on 13 May to 0310 UTC on 14 May [click to play MP4 animation]
View only this post
Read Less