Cold arctic air (surface air temperatures in the upper teens to lower 20s F) flowing across the still-warm waters of Lake Erie and Lake Ontario (sea surface temperature values as warm as the middle to upper 40s F) were 2 ingredients that helped create a major lake effect snowfall event on 18 November 2014 (VIIRS visible image with surface analysis). Storm total snowfall amounts were as high as 65 inches in Erie County, New York (NWS Buffalo Public Information Statement). GOES-13 0.63 µm visible channel images (above; click image to play animation) showed the large and well defined single-band lake effect cloud features that developed over each of the lakes. The band over Lake Erie was nearly stationary for several hours, producing snowfall rates as high as 4 inches per hour at some locations in the Southtowns of Buffalo. The stationary behavior (and very sharp northern edge, due to a “locked thermal convergence zone“) of the Lake Erie snow band was quite evident on composite radar reflectivity (below; click image to play animation; images courtesy of the College of DuPage). The formation and growth of this band benefited from a long fetch of southwesterly winds oriented along the axis of Lake Erie. Isolated negative cloud-to-ground lightning strikes were observed at 16:45 and 22:15 UTC, implying the presence of embedded pockets of thundersnow.
A comparison of Suomi NPP VIIRS 0.64 µm visible channel and 11.45 µm IR channel images at 18:17 UTC or 1:17 pm local time is shown below. The coldest cloud-top IR brightness temperature was -37º C (green color enhancement), which corresponded to a pressure of 437 hPa (or an altitude around 6 km) on the 12 UTC Buffalo NY rawinsonde report.
Comparisons of Terra and Aqua MODIS true-color Red/Green/Blue (RGB) images covering the Lake Erie/Lake Ontario region along with a high-resolution view centered on Buffalo NY are shown below.
A 15-meter resolution Landsat-8 0.59 µm panochromatic visible channel image from the SSEC RealEarth web map server (below) showed great detail to the Lake Ontario snow band as it was moving inland over the Watertown NY area at 15:45 UTC.
Looking back to the preceding nighttime hours, a toggle between Suomi NPP VIIRS 0.7 µm Day/Night Band, 3.74 µm shortwave IR, 11.45 µm IR, and 11.45-3.74 µm IR brightness temperature difference “Fog/stratus product” images at 06:54 UTC or 1:54 am local time (below) showed that the lake effect bands were already well-developed, with minimum 11.45 µm IR brightness temperatures of -30º C and colder (yellow color enhancement). Even with minimal lunar illumination — the Moon was in the Waning Crescent phase, at only 7% of full — the lake effect cloud bands features could still be seen on the Day/Night Band image.
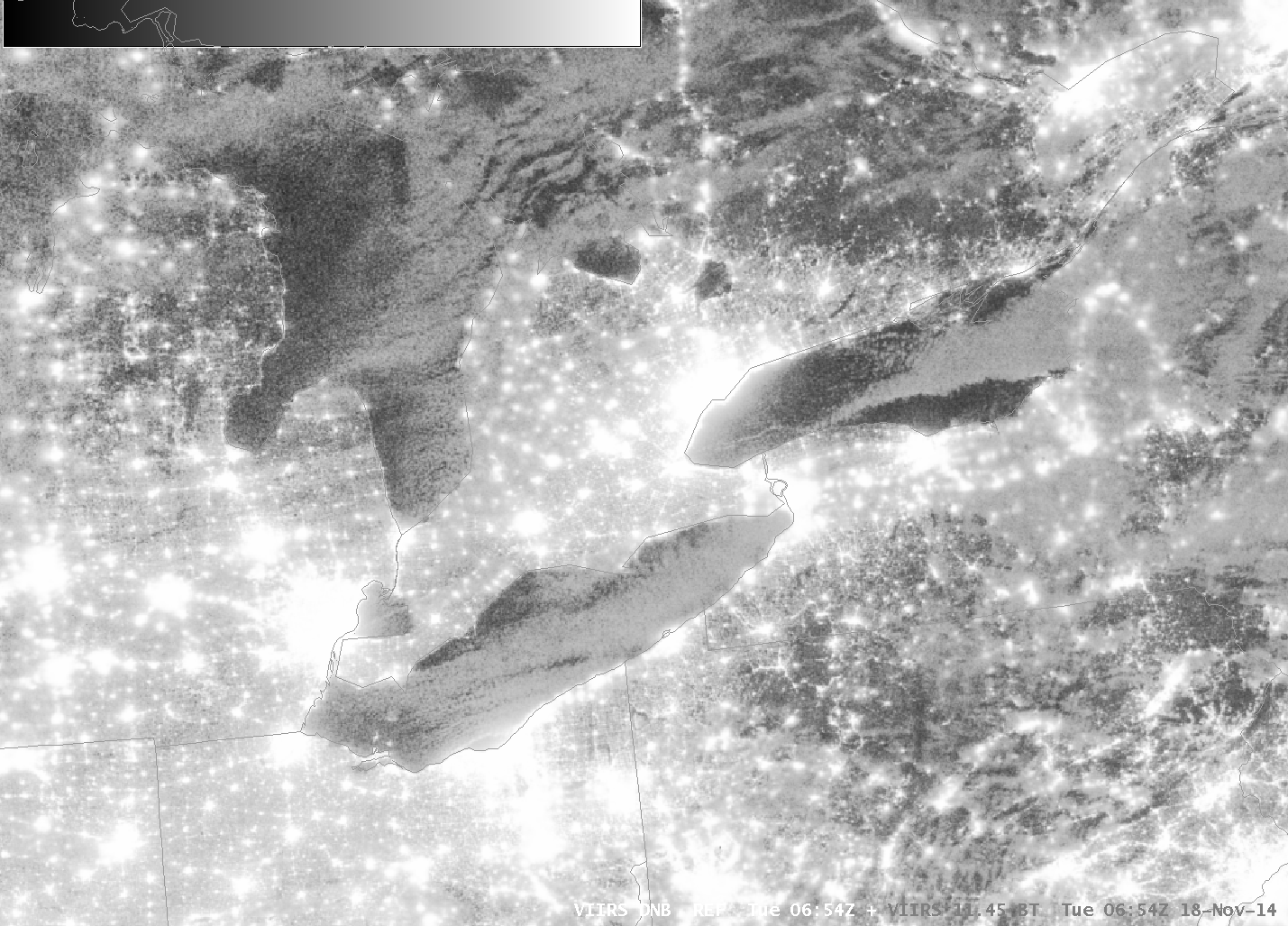
Suomi NPP VIIRS 0.7 µm Day/Night Band, 3.74 µm shortwave IR, 11.45 µm IR, and “Fog/stratus product” images
For a more in-depth discussion of this lake effect snow event, watch the VISIT Satellite Chat session.
View only this post Read Less