Blowing snow signature over northern Hudson Bay
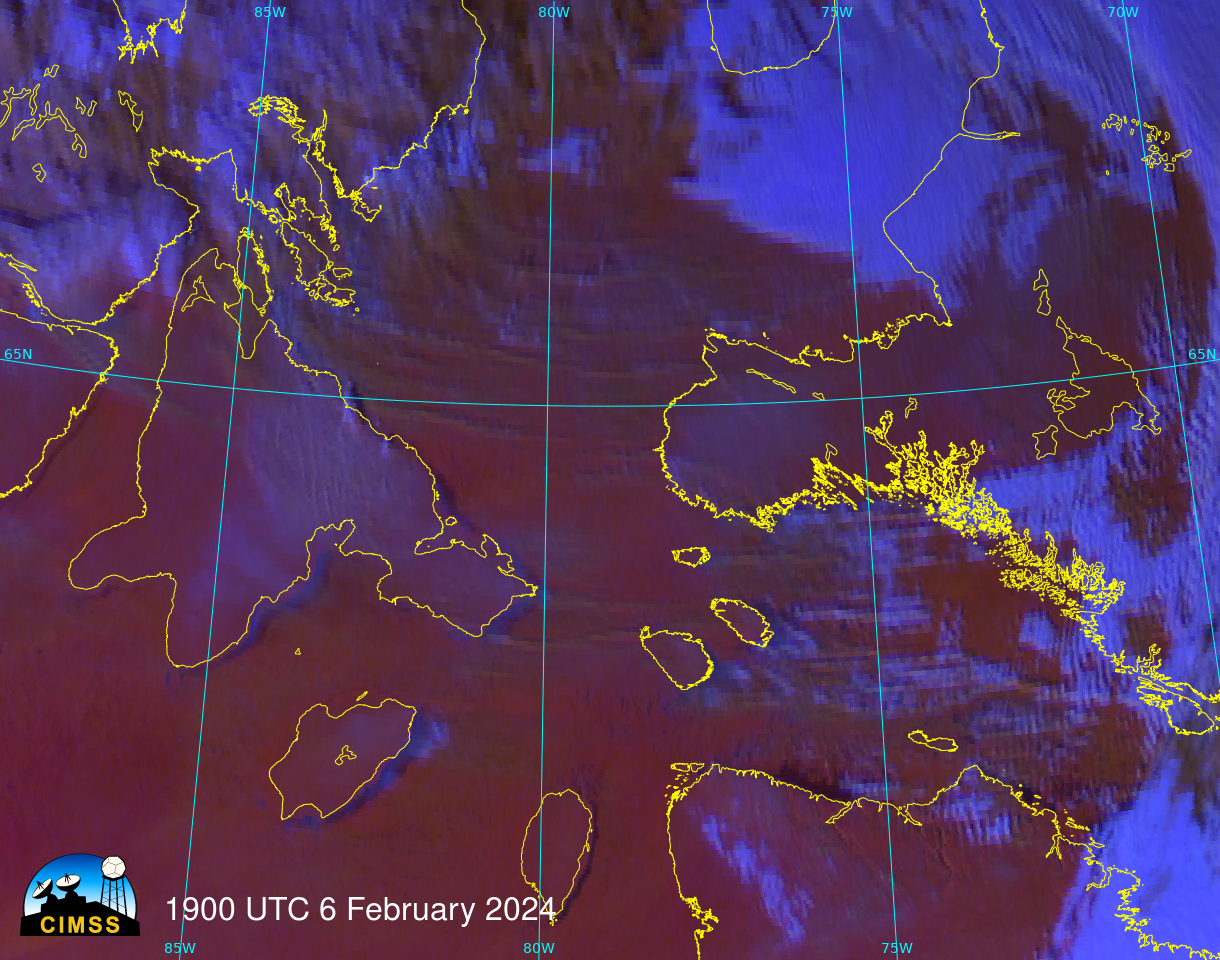
At the CIMSS Satellite Blog, we occasionally get emails from our readers. Yesterday’s (6 February 2024) email included this one: I was doing some forecasting for the Canadian Arctic today and noticed a veryimpressive blowing snow signature on satellite; not that blowing snow isuncommon up there, but rather that the... Read More