30-second imagery of severe thunderstorms over Oklahoma and Kansas
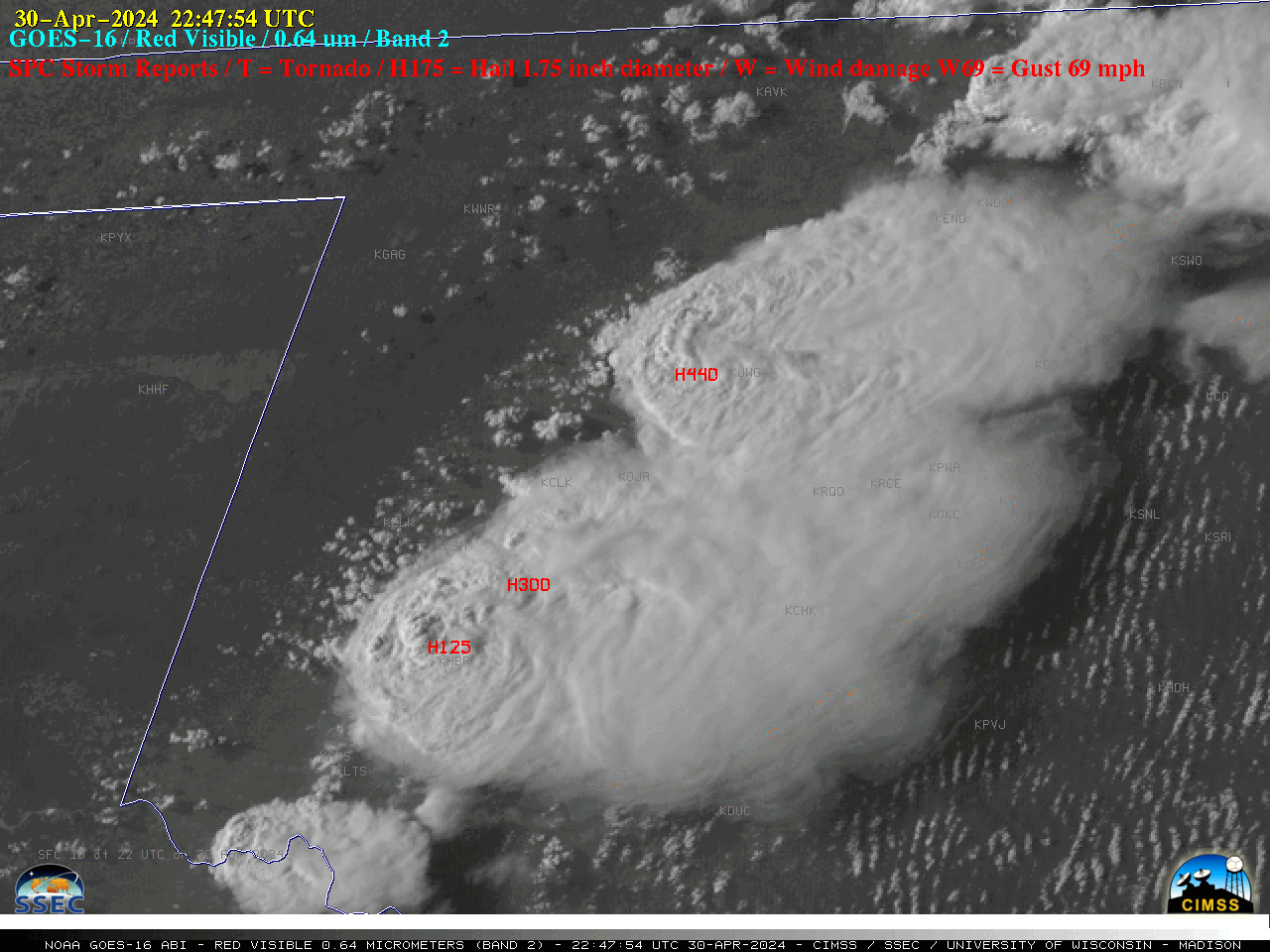
Overlapping 1-minute Mesoscale Domain Sectors provided 30-second interval GOES-16 (GOES-East) “Red” Visible (0.64 µm) images (above) — which showed thunderstorms that produced tornadoes, large hail (up to 4.4 inches in diameter) and damaging winds (SPC Storm Reports) across western Oklahoma on 30 April 2024. Pulses of overshooting tops and evidence of Above-Anvil Cirrus Plumes (reference | VISIT training | blog posts) were... Read More