NGFS detections of multiple fires in/near Los Angeles County
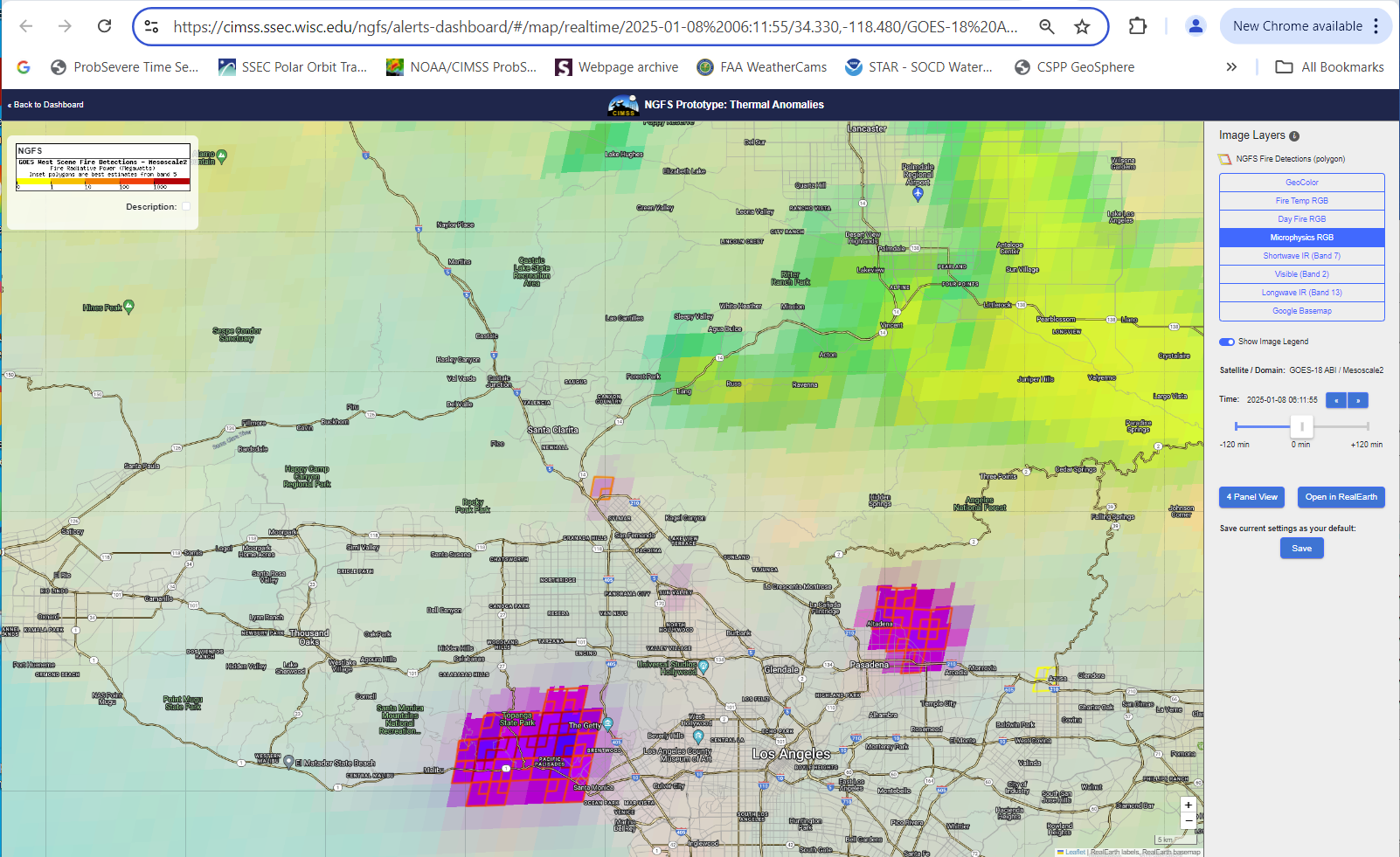
Strong Santa Ana winds and dry conditions caused multiple fires in Los Angeles area on 7-8 January 2025 (here is a blog post on the Palisades fire, for example). What information on these fires is provided by the Next-Generation Fire System (NGFS) website? The Alerts Dashboard at that site shows the latest Thermal Anomalies; when... Read More