LightningCast applied to new sensors
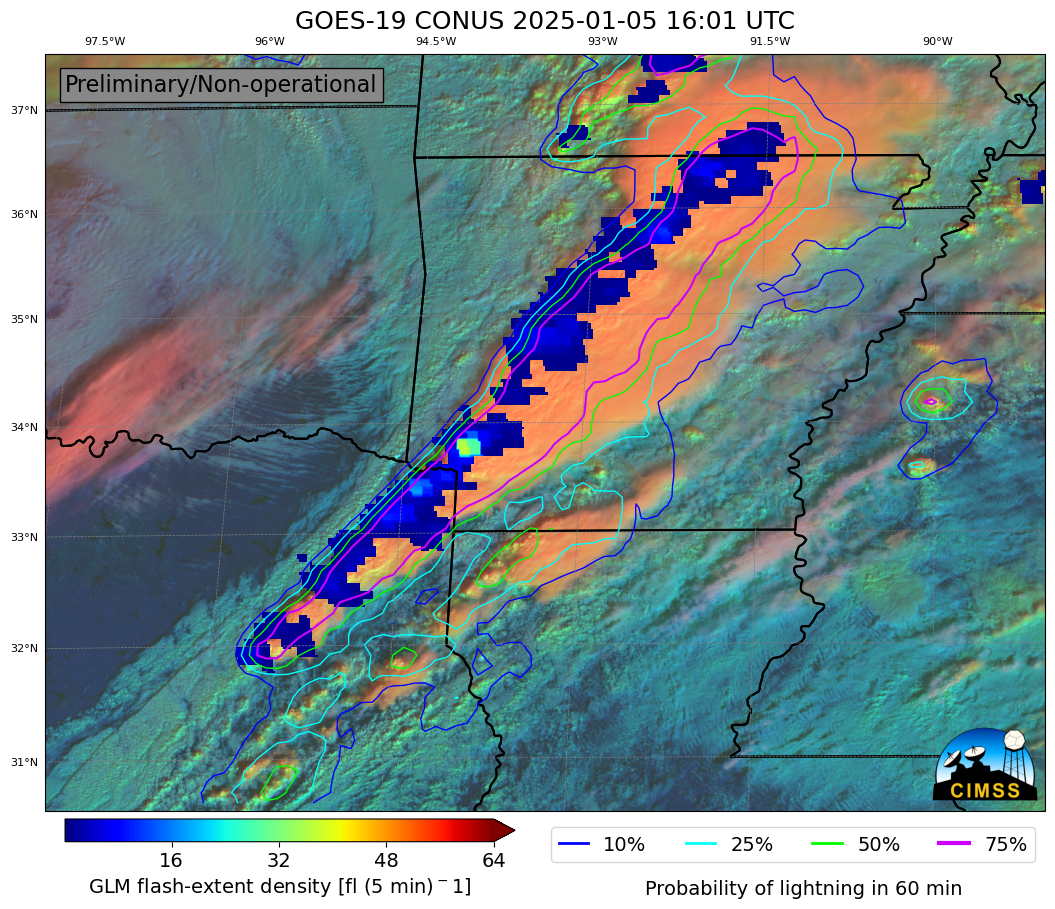
GOES-19 is slated to become the next operational GOES-East in April. Despite being trained on GOES-16 data, CIMSS and NOAA scientists have been busy evaluating the AI lightning-prediction model, LightningCast, on GOES-19 output to prepare for the operational transition and see how adaptable the model is.Overall, the LightningCast output on... Read More