Using NUCAPS profiles in lieu of missing upper air soundings
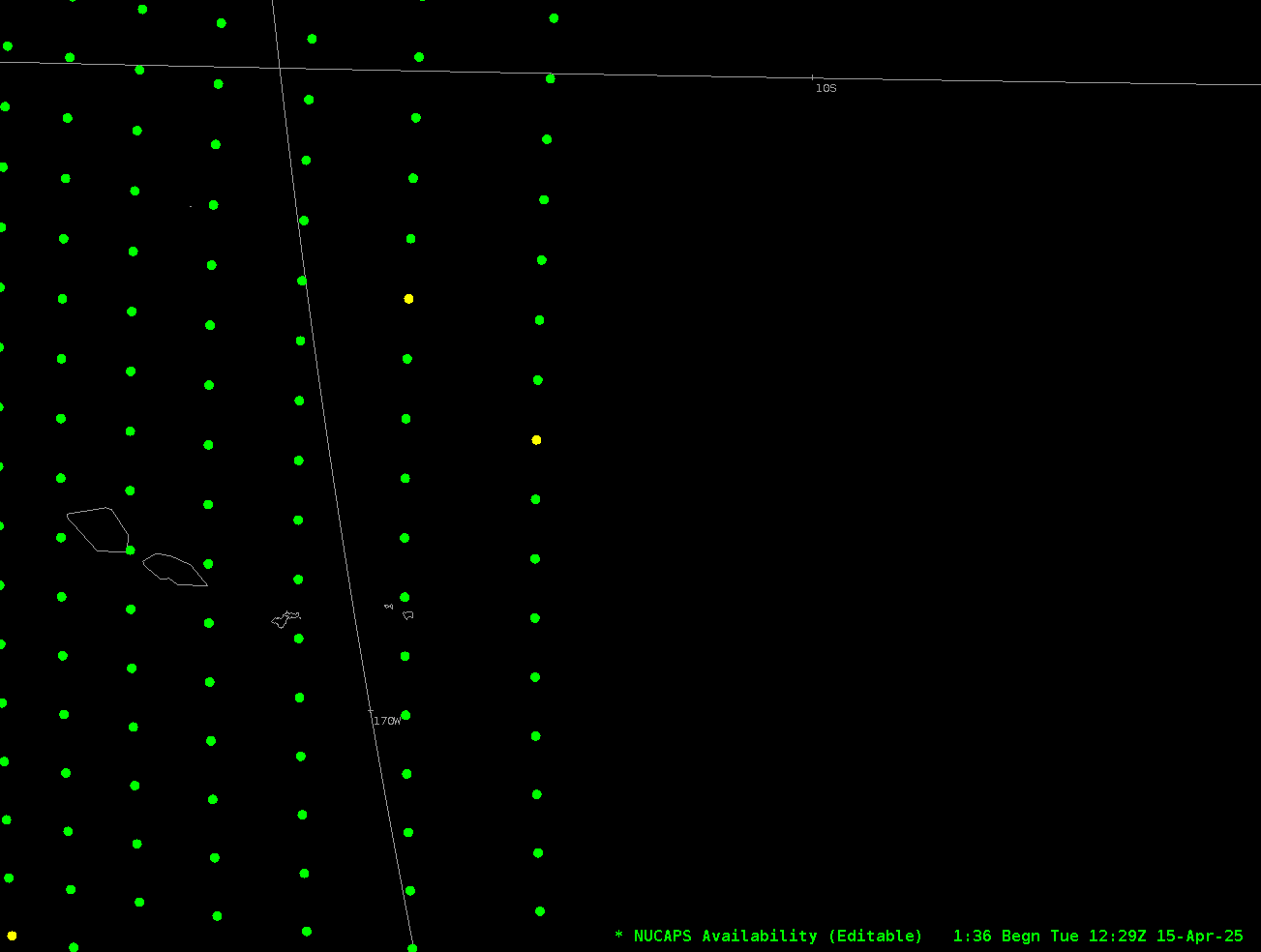
Instrument issues at the weather station in Pago Pago (related to Hydrogen generation) means that balloon launches occur only once every other day, at 0000 UTC on odd days. The sounding for 15 April/0000 UTC (1 PM Samoa Standard time on 14 April) is shown below.Two different JPSS satellites overflew... Read More