Earth formed about 4.6 billion years ago during the birth of our solar system. This date comes from meteorites and moon rocks. Studies of the surface of the moon, mercury, and other planetary bodies’ reveals that for several hundred million years after the formation of the solar system the planets were continuously bombarded by meteoric debris thus the surface of the Earth was probably repeatedly impacted and perhaps remelted by the impacts of large asteroids. This early bombardment continued until about 3.8 billion years ago.
During the next major phase of earth’s formation cooling and differentiation of the Earth’s layers occurred. The earliest Earth was probably very hot due to the release of kinetic energy during accretion, the decay of radioactive elements in its interior, and the collision that formed the moon. Partial to complete melting of the Earth’s interior allowed dense materials to sink to the center, forming an iron-nickel rich core. At the same time lighter buoyant masses of silicate-rich magma rose to the surface to form a magma ocean. The remaining material between the core and the magma ocean formed Earth’s thickest layer, called the mantle,which is composed mainly of iron, magnesium, calcium-rich silicate minerals (Figure 1).
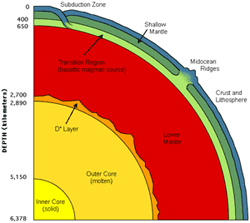
Figure 1: Cross sectional view of Earth illustrating its heterogeneous layers. (image from Beatty, J. K. and A. Chaikin, eds. The New Solar System. Massachusetts: Sky Publishing, 3rd Edition, 1990.)
Click to enlarge.
Eventually, the magma ocean would have cooled to form a thin layer of silica/aluminum-rich (with lesser amounts of iron, magnesium, calcium, sodium, and potassium) primitive crust such as is present beneath the oceans. Today there are two types of crust. Oceanic crust is composed of dense basalt and gabbro. Continental crust is less dense and has a granitic composition overall. It is probable that the Earth’s initial crust was remelted several times due to impacts with large asteroids. Continental crust would form later. As soon as the crust became cool enough not to remelt, plate tectonics must have begun (more about plate tectonics in the next lesson).
The division of the Earth’s interior into 3 distinct layers called the core, mantle, and crust is based on chemical composition. Additionally, Earth’s interior can be separated into 5 layers based on physical properties (Figure 2). Temperature and pressure relations at various depths influence the physical state of these layers. Starting from the Earth’s surface, the interior can be divided into the lithosphere, asthenosphere, mesosphere, outer core, and inner core based on physical differences.
The lithosphere is composed of the crust and upper-most portion of the mantle. In general, material of the lithosphere is solid and rigid although as temperature and pressure increase with depth the rocks get progressively easier to deform. The lithosphere is relatively very thin within ocean basins (as thin as a few km near ocean crust ridges) and considerably thicker under continents (up to more than 250 km beneath mountain belts). Beneath the lithosphere lies the asthenosphere. Here material is hot enough that it acts like a soft putty-like substance. Rocks within the asthenosphere are near their melting points and in a geophysical sense are very weak. So much so, that the overlying more rigid lithosphere can detach from the asthenosphere and move independently.
Despite high temperatures, increasing pressure within the lower mantle creates a strong solid layer called the mesosphere. The mesosphere or lower mantle is the largest layer of the Earth by volume. The outer core is mostly an iron-nickel alloy and due to extremely high temperature and that major minerals within this layer have relative low melting points it remains a molten layer. Convection currents within this layer generate Earth’s magnetic field. The inner core, also mostly an iron-nickel alloy, behaves as a metallic solid due to the immense pressure.
The Rock Cycle
The Earth is a dynamic system of interacting or intertwining cycles. One such cycle is a set of loops that involves the processes by which rock changes from one form to another. The rock cycle helps us to understand the origin of igneous, sedimentary, and metamorphic rocks and to see how these rock types are linked to one another through processes acting on and within the Earth.
Rocks can be grouped into 3 general categories: igneous, metamorphic, and sedimentary based on the way they are formed. Each of these categories contains a collection of many types of rock that differ from each other based on the composition and texture of the mineral grains they contain. Igneous rock forms from the crystallization of molten material (magma) that is generated for the most part in the upper lithosphere. Igneous rock may be classified as intrusive if the magma cools and solidifies underground. If on the other hand the molten material reaches Earth’s surface through a volcanic eruption, the resulting rock is known as extrusive igneous rock.
Once exposed to atmospheric conditions at the surface of the earth, weathering processes may cause rocks to disintegrate or decompose into loose sediment, or dissolved ions. Sediment can be transported by rivers, wave action, wind, gravity, or glacial ice, be deposited, and become buried whereby the loose grains may be compacted and cemented (lithified) and converted into clastic sedimentary rock. Chemical sedimentary rock is deposited by chemical precipitation of minerals that are held in solution. Precipitation can occur through inorganic processes such as evaporation of sea water which can result in the accumulation of rock salt, or precipitation can occur through biochemical activity such as in the formation of fossil-rich limestones composed of the buildup of sea shells or skeletal coral. Sedimentary rock may also be classified as organic such as with coal that forms from the compaction of plant material.
If buried deep enough, rock can be subjected to high temperature and / or intense pressure which may cause it to change into the third rock group called metamorphic rock. When the buried rock undergoes compressional stress or shearing forces the deformed mineral grains in the rock may become parallel to one another and have a flattened layered appearance. This planar texture is known as foliated metamorphic rock. If metamorphic rock undergoes additional heating or still higher pressure it may completely melt and once again become magma completing the rock cycle.
As illustrated in the diagram to the left, other pathways exist in the rock cycle. For example, igneous rocks can crystallize and remain beneath the Earth’s surface where they can be subjected to extreme pressure associated with mountain building events transforming them into metamorphic rock or completely remelting them to create magma. Through long erosional periods, once deeply buried metamorphic or sedimentary rock may be exposed to the Earth’s surface where it becomes loose sediment once again. Sedimentary rock can be intruded by hot magma which can cause the sedimentary rock to also melt and be incorporated into the molten mass. Over the course of Earth’s long history rocks have been formed, changed, and reformed again and again.
Geologic Time
There are two types of geologic age determinations: relative age and numerical age. Geologists in the late 18th and early 19th century studied rock layers and the fossils in them to determine relative age. William Smith was one of the most important scientists from this time who helped to develop knowledge of the succession of different fossils by studying their distribution through the sequence of sedimentary rocks in southern England. It wasn't until well into the 20th century that enough information had accumulated about the rate of radioactive decay that the age of rocks and fossils in number of years could be determined through radiometric age dating.
Relative age dating means to place events in a proper sequence or order without knowing the age in years. A geologist working with a geologic map or investigating a rock outcrop along a highway is concerned with unraveling the sequence of geologic events that occurred at a particular site in order to understand the area’s geologic history. This is an example of relative age dating. Deciphering a sequence of geologic events is done by applying fundamental principles. Four basic principles that are commonly applied include the:
1) Law of Original Horizontality: Sedimentary rock layers, and large lava flows, are initially deposited in a horizontal or nearly horizontal orientation due to gravity (Figure 4). Therefore if rock layers appear tilted to the horizon we can assume the rocks have been moved into that position by some crustal disturbance sometime after their deposition (Figure 5).
2) Law of Superposition: In an undisturbed sequence of sediments and lava flows, the layer above is younger than the layer below (Figure 4).
3) Law of Lateral Continuity: Sediments and lava flows are generally laterally continuous; if not then they are usually cut by faults. Original sedimentary layers extend to the edges of a depositional environment (such as a lake or ocean basin) until they thin or taper off or until one type of sediment laterally interfingers with another as the depositional environments change.
4) Law of Cross-Cutting Relationships: Any sediment or lava flow that is cut by a fault, another igneous body, or an erosional surface is older than the cross-cutting feature (Figure 6).
Using basic geologic principles such as the four described above, as well as the presence of distinctive fossils found in sedimentary rock layers, geologists have devised a standard geologic time scale. This is a worldwide relative time scale that can be used to correlate rocks even on different continents (Table 1).
Eon | Era | Period | Epoch | |
---|---|---|---|---|
Phanerozoic | Cenozoic | Quaternary | Holocene | |
Pleistocene | ||||
Tertiary | Pliocene | |||
Miocene | ||||
Oligocene | ||||
Eocene | ||||
Paleocene | ||||
Mesozoic | Cretaceous | |||
Jurassic | ||||
Triassic | ||||
Paleozoic | Permian | |||
* Carboniferous | Pennsylvanian | |||
Mississippian | ||||
Devonian | ||||
Silurian | ||||
Ordovician | ||||
Cambrian | ||||
Proterozoic | Precambrian (informal name) |
Vendian (or Ediacaran) | ||
Archean | ||||
Hadean |
*Outside of North America, the Pennsylvanian and Mississippian Periods are known as the Carboniferous Period.